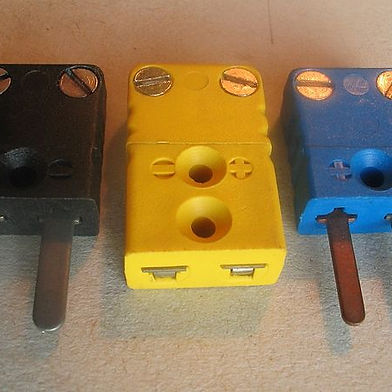
A Chunk-Based Interpretation of Thermocouple Behavior Using the Timothian Model
Thermocouples generate current from temperature differences. How can a thermocouple, such a simple combination of metals, translate thermal energy into electrical energy so effectively?
Timothian Model's Short Answer...
The junction where the metals are welded together acts as a rectifier of ambient freely moving chunks. The random jostling is organized by the mechanical rectification enough to create chunk flow and counterflow as described by this model in The Nature of Magnetism, but without generating spin of the chunks.
Details
A Chunk-Based Interpretation of Thermocouple Behavior Using the Timothian Model
Abstract
This paper offers a fully mechanical, chunk-based interpretation of thermocouple behavior as envisioned in the Timothian Model. It parallels traditional electron-based explanations—where electrical currents and voltages arise from electrons in conductors—but replaces these concepts with subatomic “chunks” flowing through atomically structured pathways. Different metals serve as “asymmetric gateways,” each with a unique arrangement of seed-based strata that leads to partial rectification of chunk flows. Temperature gradients increase or decrease these flows, creating pressure differentials (akin to voltages) and directed streams (akin to currents). The paper covers key thermoelectric phenomena (Seebeck, Peltier, and Thomson effects), saturation limits, and the roles of doping/alloying. While addressing familiar experimental observations (voltages, heating, cooling, material failures), it does so by tying them back to the Timothian stance: all forces, flows, and interactions are mechanical collisions and redistributions of subatomic chunks.
1. Introduction
In mainstream physics, a thermocouple typically consists of two dissimilar metals (e.g., Metal A and Metal B) joined at one junction, with a second reference junction located elsewhere. A temperature difference between these junctions produces an electromotive force (EMF) that can be measured as a voltage. Traditional theory interprets this through electron diffusion: hotter junctions supply more energetic electrons, driving a net current. Meanwhile, the Peltier effect describes how running a current the other way can heat or cool the junction.
The Timothian Model offers a radically different viewpoint. Instead of electrons, holes, and quantum band structures, it posits a continuous subatomic chunk medium everywhere, within which atoms exist as stable “seed plus layered spheres” configurations. Flows of these chunks drive all phenomena we usually label “electrical” or “thermal.” By reimagining conduction, magnetism, and thermoelectricity in purely mechanical terms, the Timothian Model aspires to unify everyday observations under Newtonian-like collisions—no vacuum, no quantum entanglement, and no action at a distance.
Disclaimer: The Timothian Model is an alternative framework, not a mainstream extension of conventional physics. It retains the standard experimental data (like measured voltages or temperature curves) but reinterprets them as chunk flows rather than electron behaviors.
2. Foundations of the Timothian Chunk Model
2.1 Core Postulates
Subatomic Chunks
Reality is composed of a vast zoo of subatomic chunks, varying in mass, size, and energy.
What we call “light,” “electrons,” or “photons” in standard physics becomes specific chunk species in this framework—still measurable, but now understood as mechanical lumps of mass/energy.
Atoms as Seeds with Concentric Spheres
Every atom is a seed of chunks at the center plus several concentric stratification layers. No electron “orbits” in empty space—only continuous mass distributions.
Materials differ in how these layered atoms pack together, creating unique “transparency” or “resistance” to passing chunks.
Mechanical Interactions Only
Forces are replaced by collisions, reflections, friction, and flows in the chunk medium.
Emergent “fields” (electrical, magnetic) are macro-scale manifestations of chunk flows and stratifications.
Temperature and Current as Chunk Flows
Thermal energy is random chunk motion (collisions).
Electrical current is a net, organized stream of chunks in a particular direction.
2.2 Comparison to Traditional Electron Theory
Aspect | Traditional Theory | Timothian Model |
Charge Carrier | Electrons (negatively charged), holes | Chunks of various sizes carrying mass, momentum, and potentially “charge-like” |
Conduction Mechanism | Electron drift in a conduction band | Mechanical flow of subatomic chunks through seed-based layers in each material |
Thermal Energy | Random kinetic energy of atoms/electrons | Random chunk collisions of different sizes/energies |
Voltage | Difference in electrical potential | Net chunk pressure difference or flux imbalance |
Temperature Gradient | Causes electron diffusion & thermoelectric EMF | Causes chunk flux disparities & partial rectification at metal boundaries |
Note on “Electron” Measurements: In Timothian terms, when an instrument “measures electron flow,” it’s really detecting the net movement of a particular chunk species that mainstream physics grouped under “electrons.” The data remain valid—only the interpretation changes.
3. Traditional Thermocouple Explanation (Brief Review)
Seebeck Effect: A temperature difference between two dissimilar-metal junctions yields a small voltage (EMF).
Peltier Effect: Driving current through a thermocouple junction can absorb or emit heat, depending on current direction.
Metal-Specific Coefficients: Seebeck coefficients vary by metal/alloy, making certain pairs (like K-type or J-type) more or less sensitive.
Contact Potentials: In standard view, each metal-metal contact has a contact potential. A closed loop of A–B–A–B cancels out if all junctions are at the same temperature, but differences in temperature lead to net EMF.
4. Timothian Interpretation of Thermoelectric Effects
4.1 Seebeck Effect: Rectification of Chunk Flows
Random Chunk Motion as “Heat”
Hotter regions contain more energetic collisions among chunks. The chunk flux (per unit time) is higher there, pushing more chunks through any conduction pathway.
Junctions as Partial “Valves”
At the boundary between Metal A and Metal B, each material’s layered-atom structure acts like a mechanical filter. Some chunk species cross easily from A to B, while others reflect or slow if moving B to A.
This partial rectification effect is subtle at a single junction. But in a loop with two dissimilar junctions, the net flows can add or cancel.
Temperature Imbalance = Net Flow
If both A–B junctions are at the same temperature, each “valve” effect cancels out in the loop. No net chunk flow.
If one junction is hotter, it launches more (or faster) chunks across its boundary, producing a net pressure difference around the circuit. This manifests as a measurable “voltage.”
Analogy: Flow Valve
Similar to wave refraction at an air-water boundary, or a valve letting fluid pass more easily in one direction than the other. Once you place these “valves” in a loop, a temperature difference shifts the balance, driving a current.
4.2 Peltier Effect: Heating or Cooling the Junction
Reciprocal Flow
In standard physics, passing current through a thermocouple can chill or heat the junction. Timothian logic calls it “chunk friction.”
Forcing chunks to flow in the “preferred” direction (with the valve) draws energy from the junction (cooling). Forcing them backward raises friction, heating the junction.
Directional Bias
If an external power source pushes chunks from Metal A to B in the direction the B–A boundary “likes,” collisions are fewer; net energy is carried away.
Reversing that flow can deposit more chunk energy at the boundary, akin to frictional heating.
4.3 Thomson Effect & Nonlinearities
Thomson Effect
A single conductor with a temperature gradient and a current experiences local heating or cooling.
In Timothian terms, different segments of the conductor have varying chunk densities, so friction or absorption changes as chunks flow from cooler to hotter regions (or vice versa).
Nonlinear Behavior & Material Breakdown
At very high temperatures, chunk flux can saturate the rectification boundary. The metal’s internal structure cannot handle more chunk conduction without structural breakdown.
Near phase transitions (melting, etc.), the lattice changes drastically, abruptly altering chunk transparency and making the thermocouple output jumpy or nonlinear.
5. Beyond the Basics: Key Chunk Factors
5.1 Seebeck Coefficient Sign & Magnitude
Direction of Flow: Depends on which metal more easily passes certain chunk sizes or energies. If Metal A is more transparent to larger chunks, the net current at high temperature might be A→B.
Magnitude: Related to (a) the temperature gradient (the difference in chunk flux intensities) and (b) the mismatch between each material’s chunk “valve” properties.
5.2 Alloying and Doping
Internal Lattice Modifications: Alloyed or doped metals alter the spacing, seed arrangements, and “cross-sectional channels” for chunks.
Empirical Calibration: Just as standard thermocouple manufacturers measure Seebeck coefficients, a Timothian approach would measure “chunk conduction coefficients,” reflecting how doping changes chunk permeability.
5.3 Failure Modes and Saturation
Structural Overload: Excess chunk flux (at high temp) can generate excessive collisions, friction, and meltdown.
Saturation: Before total failure, a thermocouple’s output may plateau if the junction can’t support additional flow without meltdown.
6. Comparisons to Conventional Theory
6.1 Where They Align
Temperature Difference → Voltage: Both frameworks predict a measurable EMF with temperature gradient.
Reciprocal Nature: Seebeck and Peltier effects remain two sides of the same coin.
Material Dependence: The specific composition of metals/alloys dictates the sign and scale of the observed effect.
6.2 Where They Differ
Electron vs. Chunk: Timothian physics disavows electrons as point particles, instead assigning conduction to real mechanical lumps of mass at various scales.
Rectification vs. Electron Diffusion: Traditional theory highlights chemical potential differences for electrons. Timothian sees mechanical boundary filtering—partial “valves” for chunk flows.
Saturation and Mechanical Failure: Standard theory recognizes melting or doping limits, but typically as electron scattering or band-structure collapse. Timothian focuses on direct friction and chunk collisions that physically destroy the lattice.
7. Summary & Conclusion
Thermocouple Behavior Revisited:
Two-Junction Loop: The A–B loop forms distinct mechanical “valves” at each junction. Equal temperatures cancel out flows; a temperature differential drives net chunk current (voltage).
Seebeck Sign: Governed by how each metal’s seed-based atomic layering “prefers” chunk passage.
Peltier Reciprocity: Driving chunk flow with or against the “preferred” direction yields cooling or heating due to frictional collisions at the boundary.
Thomson & Nonlinearities: Differences in chunk friction across a continuous temperature gradient can heat/cool internally; extremely high flux leads to saturations or meltdown.
Alignment with Data: Despite a new subatomic interpretation, the same lab measurements (voltage, temperature profiles) emerge. The difference lies in the mechanical viewpoint: “electrons” become chunk flows.
Implications and Outlook
Unified Mechanics: This chunk-based picture extends to all so-called “electrical” processes—batteries, circuits, magnets—under one Newtonian paradigm of collisions and flows.
Calibration in Practice: Just as standard thermocouples require empirical temperature-voltage curves, Timothian thermocouples would rely on chunk flux calibrations that can saturate under extreme conditions.
From Theory to Experiment: Future research could attempt to map how doping, alloy changes, or near-melt conditions shift chunk-based conduction coefficients, potentially providing new insight into real-world thermocouple drift or nonlinearity.
In closing, the Timothian Model sees thermocouples not as electron-driven devices but as mechanical “valves” for subatomic chunks. Temperature gradients boost chunk flux on one side, producing a net pressure difference measured as voltage. Peltier cooling and Thomson effects are similarly explained by friction-like energy transfers. While profoundly different in ontological underpinnings, this approach reproduces standard thermocouple data and phenomena—offering an alternative lens on why these everyday devices work so reliably.
Disclaimer Recap:
This interpretation does not invalidate standard thermoelectric models but re-contextualizes them under a chunk-based theory.
Experimental data remain the same; the Timothian Model challenges the idea that electrons are fundamental carriers, attributing conduction instead to mechanical lumps moving through seeds and spheres in each atom’s structure.
Whether one embraces Timothian logic or adheres to electron-based views, the measurable results of thermocouples remain a robust testament to how temperature gradients induce net flows of something—the crux lies in what we believe that “something” to be.
By reframing seemingly standard processes (like thermocouple voltages) in pure mechanical terms, the Timothian Model showcases its ambition: a single, Newtonian-like explanation spanning quantum, thermal, electrical, and cosmological scales.
Related Documents in the Full Timothian Model
Model Ontology
Preamble - The Timothian Model
The Nature of Thermodynamics
The Nature of Energy
The Nature of Pressure
The Nature of Atoms, Charge, and Chemical Bonds